Qubit Definition
A qubit, short for "quantum bit," is the fundamental unit of quantum information in quantum computing. It is the quantum analog of a classical bit, capable of existing in multiple states simultaneously. This property of superposition allows qubits to perform complex calculations much more efficiently than classical bits.
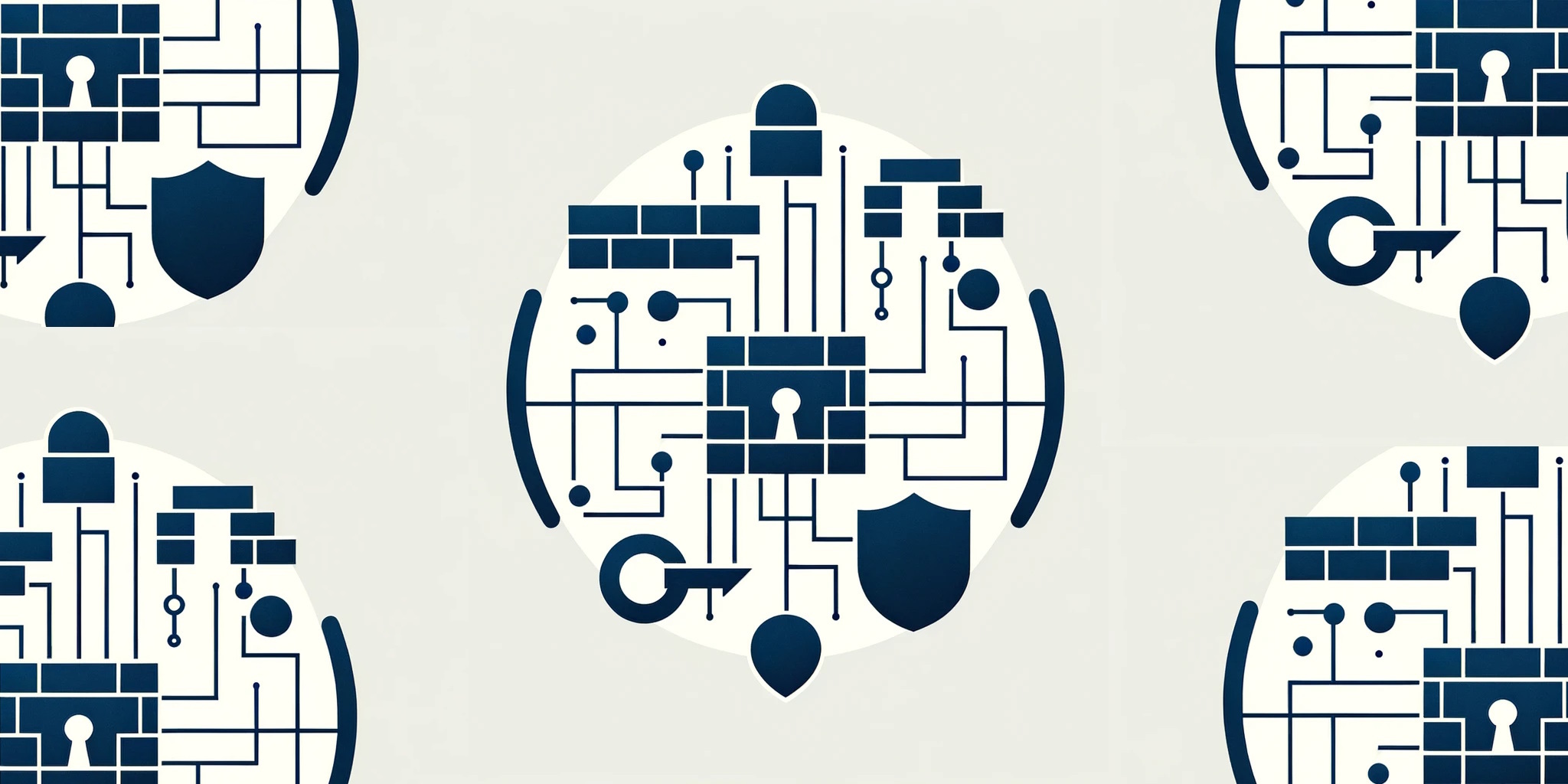
How Qubits Work
- Qubits can exist in a state of 0, 1, or both 0 and 1 simultaneously, due to quantum superposition. Superposition is a core concept in quantum mechanics where particles can exist in multiple states at the same time. In the case of qubits, this means they can be in a combination of the 0 and 1 states. This ability to be in multiple states simultaneously enables qubits to perform parallel calculations.
- Quantum entanglement is another property that qubits exhibit. Entanglement refers to a phenomenon where the state of one qubit is intrinsically linked to the state of another, even if they are physically separated. This interconnectedness allows for the creation of quantum gates, which are the building blocks of quantum computations.
- Quantum computing operations manipulate qubits using quantum gates to process vast amounts of data in parallel. These operations include computations such as quantum logic gates, measurements, and transformations. Qubits can perform these operations on an exponential scale, solving complex problems faster than classical computers.
Advancements in Qubit Technology
Physical Implementations
- There are various physical systems that can serve as qubits. These include:
- Superconducting qubits: These qubits are made from Josephson junctions, which exploit the quantum phenomena of superconductivity. They operate at extremely low temperatures and have been used by companies like IBM and Google in their quantum computing research and development.
- Trapped ion qubits: These qubits are created by trapping ions using electromagnetic fields and manipulating their internal energy states. Trapped ions have demonstrated long coherence times, making them suitable for certain types of quantum computations.
- Topological qubits: Topological qubits are a more theoretical concept that aims to use quasiparticles called anyons to store and process quantum information. These qubits have the potential of increased stability and error correction.
- Researchers are continuously exploring new materials and technologies to improve qubit performance and address the challenges of quantum decoherence, which refers to the loss or disturbance of quantum information.
Quantum Error Correction
- Quantum systems are susceptible to errors due to factors such as noise, imperfections in physical devices, and interactions with the environment. Quantum error correction techniques aim to mitigate these errors and improve the reliability of qubits and quantum computations.
- One approach to error correction is through the use of redundant qubits. By encoding quantum information into multiple qubits and performing error-detecting operations, it's possible to identify and correct errors without losing the original information.
- Quantum error correction is an active area of research, and advancements in this field are crucial for the scalability and practical implementation of quantum computers.
Quantum Supremacy and Beyond
- Quantum supremacy refers to the theoretical point at which a quantum computer can solve a problem that is intractable for classical computers. This milestone, if achieved, would demonstrate the computational power of qubits and pave the way for advancements in fields such as optimization, cryptography, and material science.
- Google claimed to achieve quantum supremacy in 2019 by performing a computation that would take a classical supercomputer thousands of years to complete. However, the debate around the definition and significance of quantum supremacy continues.
- Beyond quantum supremacy, researchers are exploring the development of fault-tolerant quantum computers capable of performing complex calculations with high accuracy and stability. This involves addressing challenges such as quantum decoherence, improving error correction techniques, and scaling up the number of qubits.
Challenges and Future Directions
Error Rates and Quantum Decoherence
- One of the major challenges in quantum computing is the high error rates associated with qubits. Quantum systems are highly sensitive to noise and disturbances, leading to errors in computations. Reducing error rates and increasing the coherence time of qubits is crucial for the practical implementation of quantum computers.
- Various techniques are being explored to address quantum decoherence, including error correction codes, fault-tolerant designs, and improved qubit manufacturing processes. Finding ways to protect qubits from environmental interactions and noise is a key focus of research.
Scalability and Quantum Interconnectivity
- Another significant challenge is scaling up the number of qubits in a quantum computer. To solve complex real-world problems, a large number of qubits is required. However, maintaining the coherence and interconnectivity of qubits becomes increasingly difficult as the number grows.
- Improving the connectivity between qubits is essential for performing complex quantum computations. Researchers are investigating methods such as improved qubit layouts, reducing crosstalk between qubits, and developing efficient interconnect technologies.
Applications of Quantum Computing
- Quantum computing has the potential to revolutionize various fields, including:
- Optimization: Quantum computers could revolutionize optimization problems, leading to more efficient solutions in areas such as logistics, finance, and supply chain management.
- Cryptography: Quantum computers could break many of the cryptographic algorithms currently in use. Therefore, the development of quantum-resistant encryption methods is crucial.
- Material Science: Quantum simulations enable researchers to study and design new materials with specific properties, accelerating the discovery of advanced materials.
- While these applications hold promise, many practical challenges need to be overcome before quantum computers can have a widespread impact.
Qubits are the building blocks of quantum computing, harnessing the principles of quantum mechanics to perform complex calculations. The property of superposition allows qubits to exist in multiple states simultaneously, while entanglement enables interconnectivity between qubits. Advancements in qubit technology, quantum error correction, and the pursuit of quantum supremacy are driving the development of practical quantum computers. However, challenges such as error rates, scalability, and developing applications still need to be addressed. Quantum computing holds potential for transformative advancements in various fields, but further research and development are necessary to unleash its full power.